Please visit the web site of the Nanonetworking Research Group (NRG) at
http://nrg.boun.edu.tr
for our Nanonetworking/Molecular Communications related research
(The content on this page is not being maintained anymore; it is kept just for historic reasons)
Literature Survey
Nanotechnology is a new, emerging field dealing with the development and manufacturing of nanoscale materials and machines. These machines, called nanomachines, are expected to have the capabilities of their higher-scale counterparts in the nanoscale. In order to perform more complex tasks, the nanomachines should be able to communicate with each other. Communication systems that enable information exchange between nanomachines are called nanonetworks [1]. The methods proposed to realize nanonetworks are composed of two groups: Traditional Communication Systems and Molecular Communication Systems. Traditional systems are systems already being used by higher scale machines (e.g., electromagnetic communication, cable-based communication, acoustic communication, and heat communication). Molecular communication systems are systems that are either being used by living organism cells or systems inspired from biological cell communication (e.g., communication via diffusion, ion signaling, microtubule – molecular motors, pheromone signaling).
In [2], five different types of cable systems are proposed. While they are similar to the cable design, the information is sent using different methods. These systems are as follows.
•Electrical cable system: Electrical signals are used similar to current wired solutions (e.g. CAT 5 cables).
•Optical cable system: Light beams are used similar to fiber optics.
•Acoustic cable system: Acoustic waves used to transmit information along the cables.
•Mechanical cable system: Information is sent by modulating the rotation of the turning of the cable.
•Chemomessenger cable system: A chemical molecule is sent along the cable.
A.Traditional Communication Systems
i.Electromagnetic Communication
Electromagnetic waves considered for communication between nanomachines are compromised of low-to-medium frequency and high-to-extremely high frequency waves. Low-to-medium frequency waves can pass through many materials including living tissue easily, which make them applicable to various scenarios. However, generating electromagnetic waves at this frequency from a nano-scale antenna requires too much energy, and the resulting signal carries very little amount of information to be useful at the receiving end. The main reason of this problem is the wavelength used in the transmission. The antenna size of an electromagnetic wave transmitter should be at least a substantial fraction of the size of the wavelength of the waves. Due to its size, a low-to-medium frequency nanoscale transmitter needs enormous amount of energy to transmit the signals. On the other hand, this kind of communication can be used to send data from micro- or macro-scale machines to nanomachines. The average energy budget of micro- and macro-machines are appropriate for low-to-medium frequency wave generation and nanoscale antennas can easily detect and decode these signals [2].
High-to-extremely high frequency signals have a higher absorption rate from materials and living tissue. Also, more transmission power is needed from the transmitter compared to lower frequency signals. Yet, an electromagnetic wave at these frequencies can easily be produced with nanoscale antennas, which decreases the required transmission power. In [2], Freitas calculates that for a nanoscale machine with a 1 pW energy budget for communication, 106 zJ is needed to transmit a single bit. This leads to a data rate limit of 103 bps for such a nanomachine.
ii. Cable-based Communication
Cable-based communication is a traditional communication paradigm that is widely used among micro- and macro-scale machines. The main advantage of any cable-based communication is the transmission medium’s isolation from the surrounding environment. This property leads to higher data rates and reliability than wireless solutions. However, the requirement for dedicated cables introduces additional design challenges, cost, and complexity. Also, the users have little to no mobility capabilities because of the cables. In case of a link failure, the faulty links must be found and fixed.
Most of the properties of cable-based communication also apply to nano-scale cable-based communication. Cables can be built using carbon nanotubes. The capability of the carbon nanotube to act as a 1-D object increases its usefulness and performance in cable-based communication. However, cable deployment makes this method’s implementation very difficult in some environments such as inside living organisms. Also, repairing links via redeployment becomes nearly impossible in such environments. As an alternative deployment procedure, the cables can be produced and deployed by the nanomachines. However, this method introduces an additional network initialization step and another, most probably wireless, communication system in this step.
iii. Acoustic Communication
Acoustic communication utilizes acoustic waves to transmit information. It is widely used as an underwater communication system since sound waves can easily propagate through water over long distances. Freitas has evaluated the use of acoustic waves for communication between nanomachines for living organisms environment in [2]. He states that the use of acoustic waves with frequencies between 10 and 100 MHz are ideal for this environment. It is calculated that acoustic communication can attain data rates up to 107 bps for distances over 10-100 micrometers (1-10 cells, assuming a cell size is approximately 10 micrometers) but consumes too much power (approximately 6000 pW) for a nanomachine. These rates are expected to be worse if the environment is selected as free space since acoustic waves travel much slower in air than in water (343 m/sec compared to 1482 m/sec). Another limiting factor for acoustic communication for nanomachines is the antenna size [1]. Similar to electromagnetic communication, the inherent small size of any potential antenna design for nanomachines decreases the usefulness of this kind of communication.
iv. Heat Communication
It is argued in [2] that heat waves can also be used for communication purposes between nanomachines. In this method, the transmitter and receiver nanomachine are immersed into a heat conductive medium and the transmitter generates heat waves to transmit information to the receiver. By modulating the frequency, amplification, and phase of the heat wave, a heat signal that carries the information similar to electromagnetic and acoustic waves is generated. However, similar to acoustic communication, this method also requires too much power to operate for a limited range; 10 pWs are required for a 1 bps transmission at 1 µm range and 1000 pWs are required for a 104 bps transmission at 100 µm range [2]. Also, this method requires the nanomachines to work in an environment that has high heat conductivity. Such an environment also amplifies the naturally occurring heat signatures already resident in the environment, which causes noise and further decreases the bit rate.
B.Molecular Communication Systems
i.Communication via Diffusion (CvD)
In CvD, the information is transmitted between the transmitter and receiver through the propagation of certain molecules via diffusion [3]. These molecules are called messenger molecules and they can be chosen as a specific type of protein, peptide, DNA sequence, or other molecular structure. At the shielding layer around the receiver, there are receptors that form chemical bonds with messenger molecules when they are in close proximity. The formation of such a chemical bond triggers an event inside the unit, and thus the transmission is received. A messenger molecule must have several properties to be suitable for this kind of communication. First, it must be intoxic to the components of the communication system (i.e., transmitter, receiver, and communication medium). It should also be easy and energy efficient to manufacture these messenger molecule at the transmitter, and its building blocks should be abundantly available in the environment where the transmitter resides.
In this communication system, information is sent using a sequence of symbols that are spread over sequential time slots with one symbol in each slot. The symbol sent by the transmitter is called the “intended symbol,” and the symbol received at the receiver is called the “received symbol.” A received symbol represents one bit information: “1” if the number of messenger molecules arriving at the receiver during a time slot exceeds a threshold, “0” otherwise. This communication system can be affected adversely from Inter Symbol Interference (ISI). Due to the diffusion dynamics, some messenger molecules may arrive after the current time slot. Thus, the receiver incorrectly decodes the received symbol of the next time slot. This error, caused by ISI, is heavily affected by the selection of the threshold value.
Moore et al. study the noise effects in a typical CvD channel in [4] and conclude that, for a reliable transmission, several molecules must be sent by the transmitter instead of a single molecule. They also develop three propagation methods for chemical communication; the first uses diffusion while the other two methods use microtubule as dedicated communication channels and antennas, respectively. Their simulation results show that such a CvD system provides 10-2 to 1 bps data rate.
In a similar work, Atakan et al. build an information theoretical model for calculating the channel capacity and error compensation of CvD [5]. According to their work, error rate is affected by chemical binding rate of the messenger molecule and the receptor, temperature, and distance between the nanomachines. They also emphasize the use of additional molecules as a means to increase the reception probabilities of the communication.
Another design criterion is power consumption. The building procedure of the messenger molecule must be a low power-cost reaction. Since a single molecule must be constructed from its building block elements for each bit in the message, increasing the number of molecules sent using a FEC-like mechanism directly affects and increases the energy cost of sending a single bit. We have developed an energy model for the CvD system that evaluates the energy consumption of the CvD system and calculates the attainable data rate based on the transmission power [6].
Various methods can be used in this view to increase the data rate of a single molecule. Instead of using one threshold, multiple thresholds can be used. Also, instead of using one type of molecule several types of molecules can be used to increase the number of bits.
ii. Microtubule and Molecular Motors
Inside every cell, there is a protein-based structure called cytoskeleton. This structure acts similar to the skeleton in higher living organisms and performs many functions for the cell. One of these functions is intracellular communication via transmitting chemical structures and organelles. The part of the cytoskeleton related to this communication process is called the Microtubule. This structure starts from an organelle called centrosome and extends to the cell membrane along the cytoskeleton to form a star-like structure where the centrosome is the center (Figure 1). Centrosome, also called the microtubule-organizing center (MTOC), is an organelle that is near the nucleus and acts as the center of the microtubular network. Each end of a given microtubule has a polarity value. The end near the centrosome has negative polarity whereas the end near the cell membrane has positive polarity. Several protein families utilize this polarity feature for movement along the microtubule. These proteins are called Motor Proteins or Molecular Motors [7].
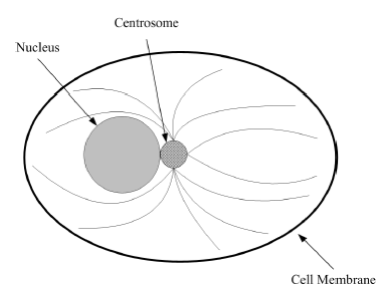
Figure 1. Microtubule Network
Two of these protein families, kinesins and dyneins, utilize microtubule as a molecular rail to carry objects between the nucleus and the cell membrane [1]. They move along the microtubule by converting chemical energy into mechanical energy by the hydrolysis of Adenosine Triphosphate (ATP) into Adenosine diphosphate (ADP) (Figure 2). Kinesin carries its loads away from the centrosome to the positive side of the microtubule. Dynein, on the other hand, brings objects to the center of the cell by moving towards the negative end of the microtubule. Each of these motor proteins consists of two parts; the head and the tail parts that are attached to the microtubule and the load that is carried, respectively. Many members exist in these protein families (e.g., humans have 45 different kinesins), each having the same head structure and a different tail depending on the type of the object it carries. Each member protein moves at a different speed. The fastest observed kinesin moves at a rate of 2-3 µm/sec. Dyneins are generally faster than kinesins, the fastest observed dynein protein moves at a speed of 14 µm/sec [7].
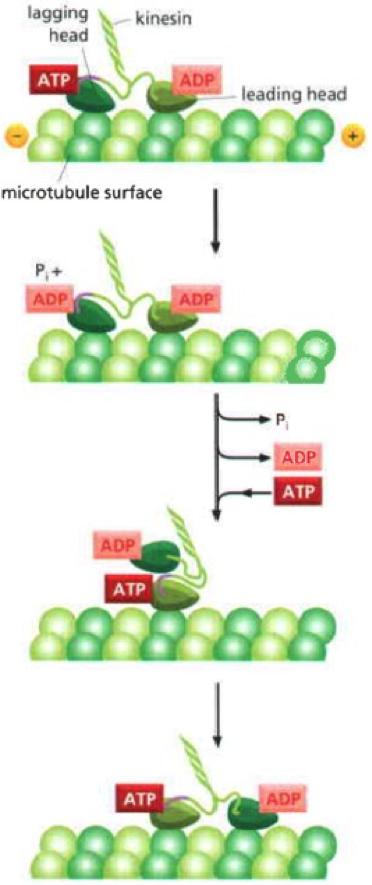
Figure 2. Kinesin Movement over Microtubule [7]
Microtubule is a structure similar to the cables in micro- and macro-scale communication. It allows communication along a predefined path with little to no interference from the surrounding environment. The polarity of the microtubule and the usage of polarity-oriented motor proteins allow a unidirectional communication along the microtubule. Using these two structures, a cable-based chemical communication system can be attained. It is considerably faster and more reliable than diffusion communication. It can be named as a Chemomessenger cable system. Microtubule can be used as an alternative to the carbon nanotubes in the cable-based communication for better bioadaptibility.
iii. Ion Signaling
Another alternative to diffusion communication in the category of chemical communication is Ion Signaling. In this method, information is sent as a wave of ion concentration change using secondary messengers. The information is encoded to ion concentration waves using classical signal modulation methods such as amplitude and frequency modulation. While different types of ions such as K+ , Na+ can be used, the most well-known and well-studied ion for this type of communication in the literature is Ca2+.
Usage of Intercellular Ca2+ Waves (ICW) in living organisms as information carriers are first noticed in the astrocyte cells, which reside in the central nervous system in animal organisms [8]. Further research revealed that various other kinds of cells (e.g., epithelial cells) also use this method for intercell communication purposes [9]. ICW is based on the relay of Ca2+ releasing secondary messengers; IP3 or ATP from the stimulated cell to the neighboring cells. When a cell is triggered by one of these messengers, the endoplasmic reticulum of the cell releases the Ca2+ ions residing inside, which leads to a high cytosolic Ca2+ concentration. After some time the ions return back to the endoplasmic reticulum and the cell returns to its former ion concentration state. Among the two different Ca2+ releasing secondary messengers, IP3 is transmitted through an internal pathway between cells while ATP is transmitted through the extracellular environment as an external pathway using diffusion (Figure 3).
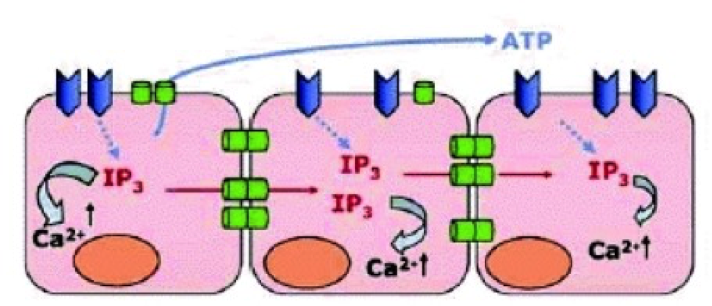
Figure 3. Intracellular Calcium Wave Propagation Methods [10]
In the internal pathway method, when a cell is stimulated, it releases IP3 molecules inside, which triggers Ca2+ release in this cell. Then, these secondary messengers pass to neighboring cells to trigger them for ion release, and thus they form the ICW. When passing between cells, IP3 molecules travel through door like structures, which are called the gap junctions.
On the other hand, in the external pathway method, after the first cell stimulation the Ca2+ concentration increases and the cell releases ATP molecules to the extracellular environment. These molecules diffuse in the environment and bind to ATP receiving receptors (e.g. P2 receptors) in the cell membrane of nearby cells. These receptors trigger the ion concentration increase in their own cells to form the ICW.
It is observed that after the first intercellular passage, the ICWs continue to spread to neighboring cells until a certain limit is reached. It has been tested that ICWs travel at speeds between 15 to 23 µm/sec and the waves can spread to 100 to 400 cells, depending on the cell type [8]. Also, according to [8], a regenerative signal model for ICWs based on observation in astrocyte cells is proposed. According to this model, when the Ca2+ concentration increases in a cell, the cell also produces Ca2+ releasing secondary messengers (IP3 or ATP depending on the pathway method) to the pathway. Thus, the signal is regenerated and amplified. While this model explains the wide spread of ICWs, it fails to explain the termination of the ICWs after some number of cells.
The gap junctions play a very important role in the realization of the ICW spread based communication. They are composed of tens to thousands of structures called connexons. A connexon is simply a tunnel to the neighboring cell where the walls of the tunnel are complex protein based molecules called connexins. If a connexon pair matches each other at two neighboring cells, they form an open gap junction channel. Gap junction channels are very small in size and allow only the transfer of ions and small water-soluble molecules; bigger molecules such as nucleic acids do not fit in these channels and cannot pass through. Connexons can also be built to form a closed gap junction channel, which blocks direct transmission between neighboring cells (Figure 4). Thus, instead of allowing the passage of the IP3 molecules to spread the ICW to the neighboring cells, closed gap junctions limit the spread of the ICW.
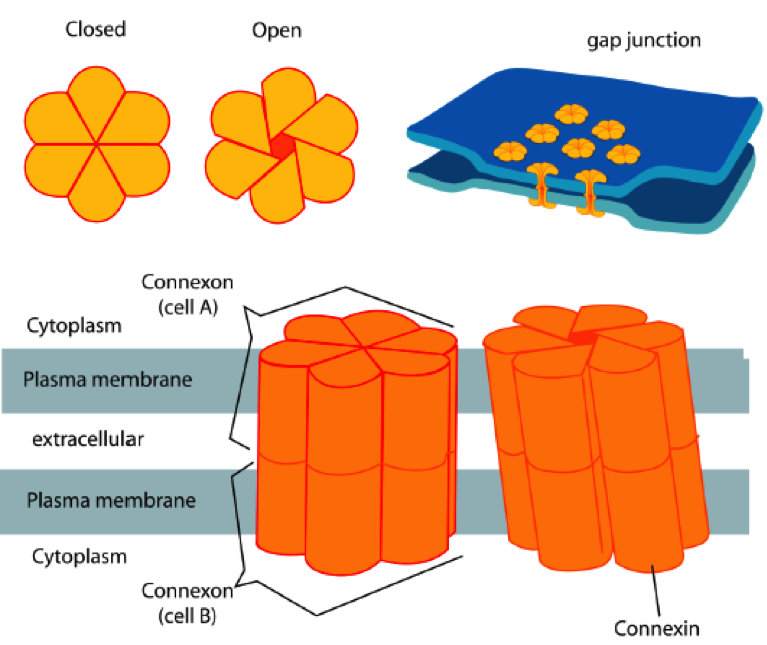
Figure 4. Gap junctions and their building blocks [11]
Each gap junction has a permeability value that regulates the transfer rate and threshold of the path. These values are not fixed and can change in seconds due to intracellular and extracellular signals (e.g. membrane potential, electromagnetic fields, temperature, pH, connexin phosphorylation, and cytolic Ca2+ concentration) [9]. The permeability of the gap junctions of a given cell can vary among different gap junctions. For example, the gap junctions on the northern part of the cell membrane can have a high permeability value while the gap junctions in the southern part of the cell membrane have a small value. Gap junctions are in a dynamic building state in the cell. New gap junctions are built while old gap junctions are destroyed. They have a half-life of a few hours, after which they are replaced with other gap junctions. This change enables the cell to alter the open and close state of its gap junctions in each iteration [7].
The external pathway based ion signaling is very similar to diffusion communication. On the other hand, ion signaling can be considered as a wired communication method when the internal pathway method is utilized in the transmission (regenerative or not). Similar to the molecular motor communication method, ion signaling using the internal pathway eliminates the use of diffusion as the propagation method of the information particles. Since diffusion is not used, propagation becomes more deterministic than diffusion communication. Noise effects from external sources are also eliminated in this method since communication takes place in the cytoplasm of the cells. An information theory based model for the propagation of Ca2+ waves is developed in [12]. However, this is currently a preliminary model and it lacks a channel capacity or error rate calculation. In ion signaling, the information wave can be relayed to a number of cells. This relay capability greatly increases the range of the communication. By altering the permeability and open/close states of the gap junctions, the ion signaling can become a broadcast, multicast, or anycast transmission instead of a unicast transmission. Nakano et al. state that signal aggregation and switching can be achieved using internal pathway ion signaling [9]. As stated above, the permeability of the gap junctions can be altered by extracellular signals. Exploiting this feature, dedicated communication channels can be established like the circuits in the circuit switching method for a given transmission.
In order to increase the data rate, different ions can be used simultaneously. Instead of sending only IP3, the nanomachines can send different water soluable molecules that trigger the release of different ions (e.g. K+, Na+) based on the type of the secondary messenger molecule type. The existence or absence of a certain ion might represent a bit, so using n different ions the ion waves can represent n bits instead of one.
iv. Pheromone Signaling
All of the molecular communication methods explained so far offer a solution for short range communication between nanomachines. However, there might be cases where nanomachines are too apart from each other so that short range communication methods are ineffective due to their range limitations. Several long range communication method are described in [13] where Gine et al. describe the use of pheromones, pollens, and light transducers for long range communications among nanomachines. The most notable of these methods is Pheromone Signaling.
Pheromones are widely used by animals and plants as chemical signals between different members of the same species. There are many different pheromones used for a variety of purposes. They usually propagate through the environment the species live in (e.g., air, water) via diffusion. In case of a transmission, the sender releases high amounts of pheromones to the environment. It only triggers a response at the receiver if the concentration of the pheromones in the environment exceeds a certain threshold. Gine et al. propose using multiple types of pheromones and classical modulation (e.g. amplitude, frequency) techniques to increase the data rate in pheromone signaling [13]. They also propose the use of special pheromone degrading enzymes to be used as a priority indicator or time-to-live parameter of the released pheromones. The most important drawback of using pheromones as a means of communication is their high delay values. It takes seconds to minutes for the pheromones to reach their destinations based on parameters such as the distance between sender and receiver, release concentration of the pheromone, and environmental parameters
2.NOVEL SCIENTIFIC VALUE
Molecular communications is an emerging research field in nanotechnology that requires interdisciplinary cooperation. There are three different approaches in nanotechnology. In the top-down approach, researchers try to scale the Microelectromechanical Systems (MEMS) down to the nanoscale. The second methodology is the bottom-up approach in which the researchers try to construct very simple nanomachines out of several atoms and molecules. In this project we adopt the third methodology, the bio-hybrid approach, which inspires from cell biology to construct nanomachines by mimicking the nature. Molecular communications is the subfield of the bio-hybrid approach that encompasses the communication features.
Communicating between cells using molecules is not a novel methodology. The research communities of molecular biology, biophysics, and medicine have been working on these mechanisms for decades. Their accumulated knowledge is an asset for us. Yet, in this immense world at the nanoscale, the approaches of these biology-related researchers have always focused on identification and treatment of diseases.
The novelty of the Molecular Communications community comes into play at this point. Rather than identifying which cells communicate using specific molecules (typically to slay those cells as in the case of cancer treatment), we try to understand, control and mimic the way in which the cells communicate. We regard the cells as processors at the nanoscale, just like the processors of the computer systems. The aim of molecular communications research is to leverage the accumulated knowledge in the biology world through the eyes of a telecommunications engineer to enable new applications.
The novelty of this project, in specific, is the study ion signaling over gap junctions as a communications method between cells. Though the cell biologists know how ion signaling works, the energy model and channel capacity are not studied. Routing via ion signaling also remains unexplored. With this project, we will contribute to molecular communications research by establishing an energy model of ion signaling. We will identify the sources of energy consumption and formulate the energy requirement to transmit a message between two cells. We will also show the data rate that can be achieved for a given energy budget. We will also explore how routing can be done in an environment where multiple cells are connected through gap junctions.
3.AIM OF THE PROJECT
The aim of this project is to study the energy model of an important method of molecular communications, ion signaling, and develop efficient routing mechanisms for nanonetworks. Nanonetworking is a new communication paradigm that focuses on the communication between nano- and/or micro- scale machines.
Ion signaling is used as an intercell communication method in closely packed cells in living tissues using ion waves as the data carriers. At each cell, the ion waves are triggered with secondary messengers moving either through the cells (called Internal Pathway) or diffusing in the environment surrounding the cells (called External Pathway).
In this project, we will first analyze the parts of the ion signaling system and develop a channel model for this system. Then, using this channel model and the components of the system, we will build an analytical energy model that governs the performance capabilities of an ion signaling system. Lastly, we will build higher level networking concepts namely, the routing mechanism upon this system for a complete communication system in molecular communication.
Our publications related with this project:
[p1]M. S. Kuran, H. B. Yilmaz, T. Tugcu, and B. Ozerman, “Energy Model for Communication via Diffusion in Nanonetworks,” Elsevier Nano Communication Networks, vol 1, no. 2, pp. 86-95, July 2010.
[p2]M. S. Kuran, H. B. Yilmaz, T. Tuğcu “Effects of Routing for Communication via Diffusion System in the Multi-node Environment” IEEE International Workshop on Molecular and Nano-scale Communications, 2011 MONACOM '11 Apr. 10 - 15 2011, Shanghai, China.
[p3]M. S. Kuran, H. B. Yilmaz, T. Tugcu, I. F. Akyildiz, “Modulation Techniques for Communication via Diffusion in Nanonetworks,” accepted to IEEE International Conference on Communications (ICC 2011), Kyoto, Japan.
References
[1]I.F. Akyildiz, F. Brunetti, C. Blazquez, “Nanonetworks: A new communication paradigm,” Computer Networks 52 (12) (2008) 2260_2279
[2]R. A. Freitas, “Nanomedicine, Volume I: Basic Capabilities,” Landes Bioscience 1999.
[3]M. Pierobon, I.F. Akyildiz, “A physical channel model for molecular communication in nanonetworks,” IEEE Journal on Selected Areas in Communications 28 (4) (2010) 602_611.
[4]M. J. Moore, T. Suda, and K. Oiwa, “Molecular Communication: Modeling Noise Effects on Information Rate,” IEEE Transactions on Nanobioscience, vol. 08, no.2, pp. 169 - 180, 2009.
[5]B. Atakan, and O. Akan, “On Channel Capacity and Error Compensation in Molecular Communication,” Transactions on Computational Systems Biology X, pp. 59 - 80, 2008.
[6]M. S. Kuran, H. B. Yilmaz, T. Tugcu, and B. Ozerman, “Energy Model for Communication via Diffusion in Nanonetworks,” Elsevier Nano Communication Networks, vol 1, no. 2, pp. 86-95, July 2010.
[7]B. Alberts, A. Johnson, J. Lewis, M. Raff, K. Roberts, and P. Walter, “Molecular Biology of the Cell,” Garland Science, 2007.
[8]E. Scemes and C. Giaume, “Astrocyte Calcium Waves: What They Are and What They Do,” Glia Journal, pp. 716 - 725 vol. 54, 2006.
[9]T. Nakano, T. Suda, M. Moore, and R. Egashira, “Molecular Communication for Nanomachines Using Intercellular Calcium Signalling,” 5th IEEE Conference on Nanotechnology, pp. 478 - 481, vol. 2, 2005.
[10]http://www.ncbi.nlm.nih.gov/bookshelf/br.fcgi?book=eurekah&part=A57070
[11]http://en.wikipedia.org/wiki/Connexon
[12]J.-Q. Liu and T. Nakano, “An information theoretic model of molecular communication based on cellular signaling,” Second Bio-Inspired Models of Network, Information and Computing Systems (Bionetics 2007), pp. 316 - 321, 2007.
[13]L. P. Gine, I. F. Akyildiz, “Molecular Communication Options for Long Range Nanonetworks,” Computer Networks Journal (Elsevier) 2009.